Stereochemistry
This entry is from Wikipedia, the leading user-contributed encyclopedia.
• Chirality
• Chiral auxiliary
• Stereochemistry
• Enantiomer
Chirality
The term chiral is used to describe an object that is non-superimposable on its mirror image. In terms of chemistry, such objects are usually molecules. The study of chiral molecules and associated phenomena is a very active area.
A molecule is chiral if it cannot be superimposed on its mirror image (see diagram); the two mirror images of such a molecule are referred to as enantiomers. A mixture of equal amounts of the two enantiomers is said to be a racemic mixture. Chirality is of interest because of its application to stereochemistry in inorganic chemistry, organic chemistry, physical chemistry, biochemistry and supramolecular chemistry.
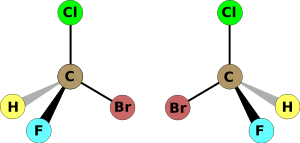
The two enantiomers of bromochlorofluoromethane
The term non-superimposable distinguishes mirror images that are superimposable, such as the letter “A” and its mirror image, from those that are not. Human hands are perhaps the most universally recognized example of chirality. The left hand is a non-superimposable mirror image of the right hand: no matter how the two hands are oriented, it is impossible for all the major features of both hands to coincide. This difference in symmetry becomes obvious if someone attempts to shake the right hand of a person using his left hand, or if a left-handed glove is placed on a right hand. Because this difference is universally known and easy to observe, many pairs of enantiomers are designated as “right- and left-handed.”
By contrast, the operation of superimposition is trivial for a non-chiral mirror image (e.g., the letter “A”). Because letters such as “A” are not three-dimensional, though, no chiral letters exist to demonstrate the contrary.
The two “handednesses” (enantiomers) of a chiral molecule are sometimes referred to as optical isomers.
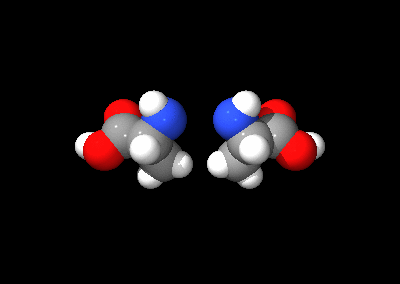
The two optical isomers of alanine
The two enantiomers of bromochlorofluoromethaneThe symmetry of a molecule (or any other object) determines whether it is chiral. A molecule is achiral (not chiral) if and only if it has an axis of improper rotation; that is, an n-fold rotation (rotation by 360°/n) followed by a reflection in the plane perpendicular to this axis which maps the molecule onto itself. (See chirality (mathematics).) A simplified rule applies to tetrahedrally-bonded carbon, as shown in the illustration: if all four substituents are different, the molecule is chiral. A chiral molecule is not necessarily asymmetric, that is, devoid of any symmetry elements, as it can have, for example, rotational symmetry.
Contents
1. History
2. Naming conventions
2.1. By configuration: R- and S-
2.2. By optical activity: (+)- and (−)-
2.3. By configuration: D- and L-
3. Types
4. Properties of enantiomers
5. Chirality in biology
6. Chirality in drugs
7. Chirality in inorganic chemistry
8. Chirality of amines
9. Chemical chirality in literature
10. References & notes
11. External links
1. History
The term optical activity derives from the interaction of chiral materials with polarized light. A solution of the (−)-form of an optical isomer rotates the plane of polarization of a beam of plane polarized light in a counterclockwise direction, vice-versa for the (+) optical isomer. The property was first observed by Jean-Baptiste Biot in 1815 [1], and gained considerable importance in the sugar industry, analytical chemistry, and pharmaceuticals. Louis Pasteur deduced in 1848 that this phenomenon has a molecular basis[2]. Artificial composite materials displaying the analog of optical activity but in the microwave regime were introduced by J.C. Bose in 1898 [3], and gained considerable attention from the mid-1980s [4].
The word “racemic” is derived from the Latin word for grape; the term having its origins in the work of Louis Pasteur who isolated racemic tartaric acid from wine.
2. Naming conventions
2.1. By configuration: R- and S-
For chemists, the R/S system is the most important nomenclature system for denoting enantiomers which does not involve a reference molecule such as glyceraldehyde. It labels each chiral center R or S according to a system by which its substituents are each assigned a priority, according to the Cahn Ingold Prelog priority rules, based on atomic number. If the center is oriented so that the lowest-priority of the four is pointed away from a viewer, the viewer will then see two possibilities: if the priority of the remaining three substitutents decreases in clockwise direction, it is labeled R (for Rectus), if it decreases in counterclockwise direction, it is S (for Sinistrus).
This system labels each chiral center in a molecule (and also has an extension to chiral molecules not involving chiral centers). It thus has greater generality than the D/L system, and can label, for example, an (R,R) isomer versus an (R,S) — diastereomers.
The R/S system has no fixed relation to the (+)/(−) system. An R isomer can be either dextrorotatory or levorotatory, depending on its exact substituents.
The R/S system also has no fixed relation to the D/L system. For example, the side-chain one of serine contains a hydroxy group, -OH. If a thiol group, -SH, were swapped in for it, the D/L labeling would, by its definition, not be affected by the substitution. But this substitution would invert the molecule’s R / S labeling, due to the fact that the CIP priority of CH2OH is lower than that for CO2H but the CIP priority of CH2SH is higher than that for CO2H.
For this reason, the D/L system remains in common use in certain areas of biochemistry, such as amino acid and carbohydrate chemistry, because it is convenient to have the same chiral label for all of the commonly-occurring structures of a given type of structure in higher organisms. In the D/L system, they are all L; in the R / S system, they are mostly S but there are some common exceptions.
2.2. By optical activity: (+)- and (−)-
An enantiomer can be named by the direction in which it rotates the plane of polarized light. If it rotates the light clockwise (as seen by a viewer towards whom the light is traveling), that enantiomer is labeled (+). Its mirror-image is labeled (−). The (+) and (−) isomers have also been termed d- and l-, respectively (for dextrorotatory and levorotatory). This labeling is easy to confuse with D- and L-.
2.3. By configuration: D- and L-
An optical isomer can be named by the spatial configuration of its atoms. The D/L system does this by relating the molecule to glyceraldehyde. Glyceraldehyde is chiral itself, and its two isomers are labeled D and L. Certain chemical manipulations can be performed on glyceraldehyde without affecting its configuration, and its historical use for this purpose (possibly combined with its convenience as one of the smallest commonly-used chiral molecules) has resulted in its use for nomenclature. In this system, compounds are named by analogy to glyceraldehyde, which generally produces unambiguous designations, but is easiest to see in the small biomolecules similar to glyceraldehyde. One example is the amino acid alanine: alanine has two optical isomers, and they are labeled according to which isomer of glyceraldehyde they come from. Glycine, the amino acid derived from glyceraldehyde, incidentally, has no optical activity as it is not chiral (achiral). Alanine, however, is chiral.
The D/L labeling is unrelated to (+)/(−); it does not indicate which enantiomer is dextrorotatory and which is levorotatory. Rather, it says that the compound’s stereochemistry is related to that of the dextrorotatory or levorotatory enantiomer of glyceraldehyde. Nine of the nineteen L-amino acids commonly found in proteins are dextrorotatory (at a wavelength of 589 nm), and D-fructose is also referred to as levulose because it is levorotatory.
The dextrorotatory isomer of glyceraldehyde is in fact the D isomer, but this was a lucky guess.[citation needed] At the time this system was established, there was no way to tell which configuration was dextrorotatory.[citation needed] (If the guess had turned out wrong, the labeling situation would now be even more confusing.)[citation needed]
A rule of thumb for determining the D/L isomeric form of an amino acid is the “CORN” rule. The groups:
COOH, R, NH2 and H (where R is a variant carbon chain)
are arranged around the chiral center carbon atom. Sighting with the hydrogen atom away from the viewer, if these groups are arranged clockwise around the carbon atom, then it is the D-form. If counter-clockwise, it is the L-form.
3. Types
Most commonly, chiral molecules have point chirality, centering around a single atom, usually carbon, which has four different substituents. The two enantiomers of such compounds are said to have different absolute configurations at this center. This center is thus stereogenic (i.e., a grouping within a molecular entity that may be considered a focus of stereoisomerism), and is exemplified by the α-carbon of amino acids. A molecule can have multiple chiral centers without being chiral overall if there is a symmetry element (a mirror plane or inversion center) which relates the two (or more) chiral centers. Such a molecule is called a meso compound. It is also possible for a molecule to be chiral without having actual point chirality. Commonly encountered examples include 1,1′-bi-2-naphthol (BINOL) and 1,3-dichloro-allene which have axial chirality, and (E)-cyclooctene which has planar chirality.
It is important to keep in mind that molecules which are dissolved in solution or are in the gas phase usually have considerable flexibility and thus may adopt a variety of different conformations. These various conformations are themselves almost always chiral. However, when assessing chirality, one must use a structural picture of the molecule which corresponds to just one chemical conformation – the most symmetric conformation possible.
When the optical rotation for an enantiomer is too low for practical measurement it is said to exhibit cryptochirality.
Even isotopic differences must be considered when examining chirality. Replacing one of the two 1H atoms at the CH2 position of benzyl alcohol with a deuterium (²H) makes that carbon a stereocenter. The resulting benzyl-α-d alcohol exists as two distinct enantiomers, which can be assigned by the usual stereochemical naming conventions. The S enantiomer has [α]D = +0.715°.[5]
4. Properties of enantiomers
Enantiomers are identical with respect to ordinary chemical reactions, but differences arise when they are in the presence of other chiral molecules or objects. Different enantiomers of chiral compounds often taste and smell different and have different effects as drugs – see below.
One chiral ‘object’ that interacts differently with the two enantiomers of a chiral compound is circularly polarised light: An enantiomer will absorb left- and right-circularly polarised light to differing degrees. This is the basis of circular dichroism (CD) spectroscopy. Usually the difference in absorptivity is relatively small (parts per thousand). CD spectroscopy is a powerful analytical technique for investigating the secondary structure of proteins and for determining the absolute configuratations of chiral compounds, particularly transition metal complexes. CD spectroscopy is replacing in polarimetry as a method for characterising chiral compounds, although the latter is still popular with sugar chemists.
5. Chirality in biology
Many biologically-active molecules are chiral, including the naturally-occurring amino acids (the building blocks of proteins), and sugars. Interestingly, in biological systems most of these compounds are of the same chirality: most amino acids are L and sugars are D. Typical naturally occurring proteins, made of L amino acids, are known as left-handed proteins, while D amino acids produce right-handed proteins.
The origin of this homochirality in biology is the subject of much debate. Most scientists believe that Earth’s life’s choice of chirality was purely random, and that it is possible that the chemistry of some alien forms of carbon-based life – assuming it exists – may have opposite chirality. However, a few scientists are looking for fundamental reasons that favor the chirality as here on earth, such as the weak nuclear force.[1]
Enzymes, which are chiral, often distinguish between the two enantiomers of a chiral substrate. Imagine an enzyme as having a glove-like cavity which binds a substrate. If this glove is right handed, then one enantiomer will fit inside and be bound while the other enantiomer will have a poor fit and is unlikely to bind.
D-form amino acids tend to taste sweet, whereas L-forms are usually tasteless. Spearmint leaves and caraway seeds respectively contain L-carvone and D-carvone – enantiomers of carvone. These smell different to most people because our olfactory receptors also contain chiral molecules which behave differently in the presence of different enantiomers.
6. Chirality in drugs
Many chiral drugs must be made with high enantiomeric purity due to potential side-effects of the other enantiomer. (The other enantiomer may also merely be inactive.)
• Thalidomide: Thalidomide is racemic. One enantiomer is effective against morning sickness while the other is teratogenic. Unfortunately, in this case administering just one of the enantiomers to a pregnant patient does not help to as the two enantiomers are readily interconverted in vivo. Thus, if a person is given either enantiomer, both the D and L isomers will eventually be present in the patient’s serum.
• Ethambutol: Whereas one enantiomer is used to treat tuberculosis, the other causes blindness.
• Naproxen: One enantiomer is used to treat arthritis pain, but the other causes liver poisoning with no analgesic effect.
• Steroid receptor sites also show stereoisomer specificity.
• Penicillin’s activity is stereoselective. The antibiotic only works on peptide links of D-alanine which occur in the cell walls of bacteria – but not in humans. The antibiotic can kill only the bacteria, and not us, because we don’t have these D-amino acids.
7. Chirality in inorganic chemistry
Many coordination compounds are chiral; for example the well-known [Ru(2,2′-bipyridine)3]2+ complex in which the three bipyridine ligands adopt a chiral propeller-like arrangement [6]. In this case, the Ru atom may be regarded as a stereogenic centre, with the complex having point chirality. The two enantiomers of complexes such as [Ru(2,2′-bipyridine)3]2+ may be designated as Λ (left-handed twist of the propeller described by the ligands) and Δ (right-handed twist). Hexol is a chiral cobalt complex which was first investigated by Alfred Werner. Resolved hexol is significant as being the first compound devoid of carbon to display optical activity.
8. Chirality of amines
Tertiary amines (see image) are chiral in a way similar to carbon compounds: the nitrogen atom bears four distinct substituents counting the lone pair. However, the energy barrier for the inversion of the stereocenter is typically about 30 kJ/mol, which means that the two stereoisomers are rapidly interconverted at room temperature. As a result, amines such as NHRR’ cannot be resolved optically and NRR’R” can only be resolved when the R, R’, and R” groups are constrained in cyclic structures.
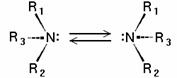
9. Chemical chirality in literature
Although little was known about chemical chirality in the time of Lewis Carroll, his work Through the Looking-glass contains a prescient reference to the differing biological activities of enantiomeric drugs: “Perhaps Looking-glass milk isn’t good to drink,” Alice said to her cat.
In James Blish’s Star Trek novella Spock Must Die! the tachyon ‘mirrored’ Mr Spock is later discovered to have stolen chemical reagents from the medical bay and to have been using them to convert certain amino acids to opposite-chirality isomers, since the mirrored Mr Spock’s metabolism is reversed and hence must process the opposite polarity of these isomers.
10. References & notes
1. Lakhtakia, A. (ed.) (1990). Selected Papers on Natural Optical Activity (SPIE Milestone Volume 15). SPIE.
2. Pasteur, L. (1848). “Researches on the molecular asymmetry of natural organic products, English translation of French original, published by Alembic Club Reprints (Vol. 14, pp. 1-46) in 1905, facsimile reproduction by SPIE in a 1990 book”.
3. Bose, J. C. (1898). “On the rotation of plane of polarisation of electric waves by a twisted structure, Proc. R. Soc. Lond. (Vol. 63, pp. 146-152), facsimile reproduction by Wiley in a 2000 book”.
4. Ernest L. Eliel and Samuel H. Wilen (1994). The Sterochemistry of Organic Compounds. Wiley-Interscience.
5. Streitwieser, A., Jr.; Wolfe, J. R., Jr.; Schaeffer, W. D. (1959). “Stereochemistry of the Primary Carbon. X. Stereochemical Configurations of Some Optically Active Deuterium Compounds”. Tetrahedron 6: 338–344.
6. Alex von Zelewsky (1996). Stereochemistry of Coordination Compounds, Wiley.
11. External links
• http://www.chemguide.co.uk/basicorg/isomerism/optical.html#top
• Nomenclature and Symbolism for Amino Acids and Peptides
• Designating the Configuration of Chiral Centers
Chiral auxiliary
A chiral auxiliary is a chemical compound or unit that is temporarily incorporated into an organic synthesis so that it can be carried out asymmetrically with the selective formation of one of two enantiomers [1] [2] . Chiral auxiliaries are optically active compounds and introduce chirality in otherwise racemic compounds. The temporary stereocenter then forces the asymmetric formation of a second stereocenter using steric hindrance or directing groups to determine chirality. After the creation of the second stereocenter the original auxiliary can be removed in a third step and recycled.
Chiral auxiliaries were introduced by E.J. Corey in 1978 with chiral 8-phenylmenthol and by B.M. Trost in 1980 with chiral mandelic acid. The menthol compound is difficult to prepare and an alternative is trans-2-phenyl-1-cyclohexanol introduced by J. K. Whitesell in 1985.
Examples
A good example of chiral auxiliaries are the class of Evans auxiliaries comprising oxazolidinones substituted at the 4 and 5 positions. Through steric hindrance, the substituents direct the direction of substitution of various groups. The auxiliary is subsequently removed e.g. through hydrolysis.
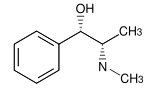
Pseudoephedrine
PseudoephedrineBoth (R,R)- and (S,S)-pseudoephedrine are used as a chiral auxiliary.[3] Pseudoephedrine is reacted with a carboxylic acid, acid anhydride, or acyl chloride to give a pseudoephedrine amide.
The α-proton of the carbonyl compound is easily deprotonated by a non-nucleophilic base to give the enolate, which can further react. The configuration of the addition compound, such as with an alkyl halide, is directed by the methyl group. Thus, any addition product will be anti to the methyl and syn with the hydroxyl group.
The pseudoephedrine chiral auxiliary is subsequently removed by cleaving the amide bond with an appropriate nucleophile.
In one study the chiral auxiliary is (-)-ephedrine [4]:
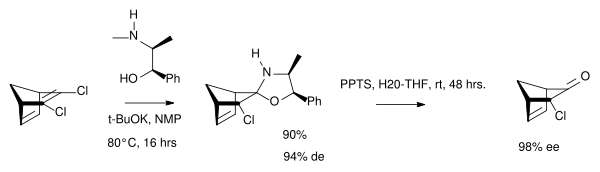
References
1. Compendium of chiral auxiliary applications (ed.: Roos, G.), Academic Press, New York, 2002.
2. Recommended concise introduction: Chiral auxiliaries – principles and recent applications; Gnas, Y.; Glorius, F. Synthesis 2006, 1899.
3. Myers, A. G., et al, Pseudoephedrine as a Practical Chiral Auxiliary for the Synthesis of Highly Enantiomerically Enriched Carboxylic Acids, Alcohols, Aldehydes, and Ketones, J. Am. Chem. Soc., 1997, 119, 6496-6511.
4. Chiral Polycyclic Ketones via Desymmetrization of Dihaloolefins Giuseppe Borsato, Anthony Linden, Ottorino De Lucchi, Vittorio Lucchini, David Wolstenholme, and Alfonso Zambon J. Org. Chem.; 2007; 72(11) pp 4272-4275.
Stereochemistry
Stereochemistry, a subdiscipline of chemistry, involves the study of the relative spatial arrangement of atoms within molecules. An important branch of stereochemistry is the study of chiral molecules.
Stereochemistry is a hugely important facet of chemistry and the study of stereochemical problems spans the entire range of this subject: organic, inorganic, biological, physical and supramolecular chemistry.
Stereochemistry includes methods for determining and describing these relationships; the effect on the physical or biological properties these relationships impart upon the molecules in question, and the manner in which these relationships influence the reactivity of the molecules in question (dynamic stereochemistry).
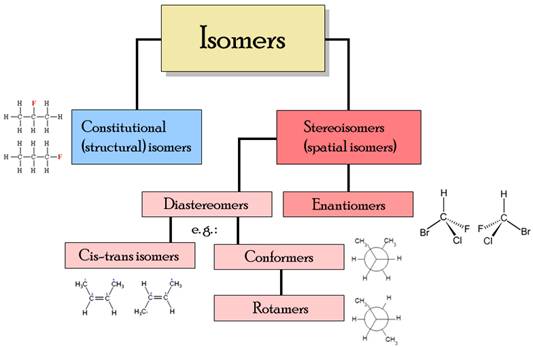
The different types of isomers. Stereochemistry focuses on stereoisomers
Louis Pasteur could rightly be described as the first stereochemist, having observed in 1849 that salts of tartaric acid collected from wine production vessels could rotate plane polarized light, but that salts from other sources did not. This property, the only physical property in which the two types of tartrate salts differed, is due to optical isomerism. In 1874, Jacobus Henricus van ‘t Hoff and Joseph Le Bel explained optical activity in terms of the tetrahedral arrangement of the atoms bound to carbon.
One of the most infamous demonstrations of the significance of stereochemistry is the thalidomide disaster. Thalidomide is a drug, first prepared in 1957 in Germany, prescribed for treating morning sickness in pregnant women. The drug however was discovered to cause deformation in babies. It was discovered that one optical isomer of the drug was safe while the other had teratogenic effects, causing serious genetic damage to early embryonic growth and development. In the human body, thalidomide undergoes racemization: even if only one of the two stereoisomers is ingested, the other one is produced. Thalidomide is currently used as a treatment for leprosy and must be used with contraceptives in women to prevent pregnancy related deformations. This disaster was a driving force behind requiring strict testing of drugs before making them available to the public.
Cahn-Ingold-Prelog priority rules are part of a system for describing a molecule’s stereochemistry. They rank the atoms around a stereocenter in a standard way, allowing the relative position of these atoms in the molecule to be described unambiguously.
Enantiomer
In chemistry, enantiomers are stereoisomers that are nonsuperimposable complete mirror images of each other, much as one’s left and right hands are “the same” but opposite.
Enantiomers have, when present in a symmetric environment, identical chemical and physical properties except for their ability to rotate plane-polarized light by equal amounts but in opposite directions. A mixture of equal parts of an optically active isomer and its enantiomer is termed racemic and has a net rotation of plane-polarized light of zero.
Two symmetrical enantiomers often do have different chemical properties related to other substances that are also enantiomers. Since many molecules in the bodies of living beings are enantiomers themselves, there is often a marked difference in the effects of two symmetrical enantiomers on living beings, including human beings.
1. Naming conventions
There are several conventions used for naming chiral compounds, all displayed as a prefix before the chemical name of the substance:
• (+)- versus (−)-; also written d- vs. l-
– based on the substance’s ability to rotate polarized light.
• D- vs. L-
– based on the actual geometry of each enantiomer, with the version synthesized from naturally occurring (+)-glyceraldehyde being considered the D- form.
• (R)- vs. (S)-
– based on the actual geometry of each enantiomer, using the Cahn-Ingold-Prelog priority rules to classify the form. Molecules with multiple stereogenic centers will have a corresponding number of letters; e.g. natural (+)-α-tocopherol is R,R,R-α-tocopherol.
The (+)- vs. (−)- convention is the only one based on optical properties. The other two conventions are based on the actual geometry of each enantiomer. There is no correspondence between any convention. In nature, many chiral substances are only produced in one optical form, while (most) man-made chiral substances are racemic mixtures. The purity of enantiomers can be determined by optical rotation.
2. More definitions
• Any non-racemic chiral substance is called scalemic.
• A chiral substance is enantiopure or homochiral when only one of two possible enantiomers is present.
• A chiral substance is enantioenriched or heterochiral when an excess of one enantiomer is present but not to the exclusion of the other.
• Enantiomeric excess or ee is a measure for how much of one enantiomer is present compared to the other. For example, in a sample with 40% ee in R, the remaining 60% is racemic with 30% of R and 30% of S, so that the total amount of R is 70%.
3. Enantioselective preparations
Several strategies exist for the preparation of enantiopure compounds. This first method is the separation of a racemic mixture into its isomers, a process called chiral resolution. Louis Pasteur in his pioneering work was able to isolate the isomers of tartaric acid because they crystallize from solution as crystals each with a different symmetry. A less common method is by enantiomer self-disproportionation.
Other methods are chiral pool synthesis: using chiral starting material and maintaining the chirality; asymmetric induction, the use of chiral auxiliaries, chiral reagents, and chiral catalysts to favor the reaction of one diastereomer over another, and the use of biocatalysts.
Enantioconvergent synthesis is the the synthesis of one enantiomer from a racemic precursor molecule utilizing both enantiomers.
4. Enantiopure medications
Advances in industrial chemical processes have made it economical for pharmaceutical manufacturers to take drugs that were originally marketed in racemic form and market the individual enantiomers, each of which may have unique properties. For some drugs, such as zopiclone, only one enantiomer (eszopiclone) is active; the FDA has allowed such once-generic drugs to be patented and marketed under another name. In other cases, such as ibuprofen, it is not economically feasible to isolate a single enantiomer from a racemic mixture or to synthesize just the active one, and therefore a racemic mixture is marketed, with an essentially doubled recommended dose.
Examples of racemic mixtures and the corresponding single-enantiomer products that have been marketed include:
• Amphetamine (Benzedrine; street amphetamine is also racemic) and dextroamphetamine (Dexedrine)
• Bupivacaine (Marcain) and levobupivacaine (Chirocaine)
• Cetirizine (Zyrtec / Reactine) and levocetirizine (Xyzal)
• Citalopram (Celexa / Cipramil) and escitalopram (Lexapro / Cipralex)
• Methylphenidate (Ritalin) and dexmethylphenidate (Focalin)
• Modafinil (Provigil) and armodafinil (Nuvigil)
• Ofloxacin (Floxin) and levofloxacin (Levaquin)
• Omeprazole (Prilosec) and esomeprazole (Nexium)
• Salbutamol (Ventolin) and levalbuterol (Xopenex)
• Zopiclone (Imovane) and eszopiclone (Lunesta)
Thalidomide is an example of a racemic drug, in which one enantiomer produces a desirable antiemetic effect, whereas the other is toxic and produces a teratogenic side-effect. However, the enantiomers are converted into each other in vivo, so chemical processes may not be used to mitigate its toxicity.